Studying the physiological mechanisms of cognitive functions requires understandings not only the responses of single neurons to external stimuli but also circuit computations at the level of networks of neurons during cognitive processing. To understand the ‘syntax’ underlying neuronal communications, methods for monitoring and quantifying cooperative neuronal activities during cognition are required. To this end, we have been performing large-scale high-density recordings of local circuits with multi-channel silicon probes, enabling the observation of simultaneous neuronal firing activities in up to 100 neurons, as well as local field potentials in behaving animals. In addition, we are developing a new technique that combines large-scale recording and targeted simultaneous optogenetic stimulations of specific cells, such as dopaminergic neurons, to clarify the role of the different types of neurons in network processing, in freely behaving mice. Taking advantage of these methods, we try to decipher circuit computations within local and between the inter-regional networks during reward-related cognitions such as decision making and working memory.
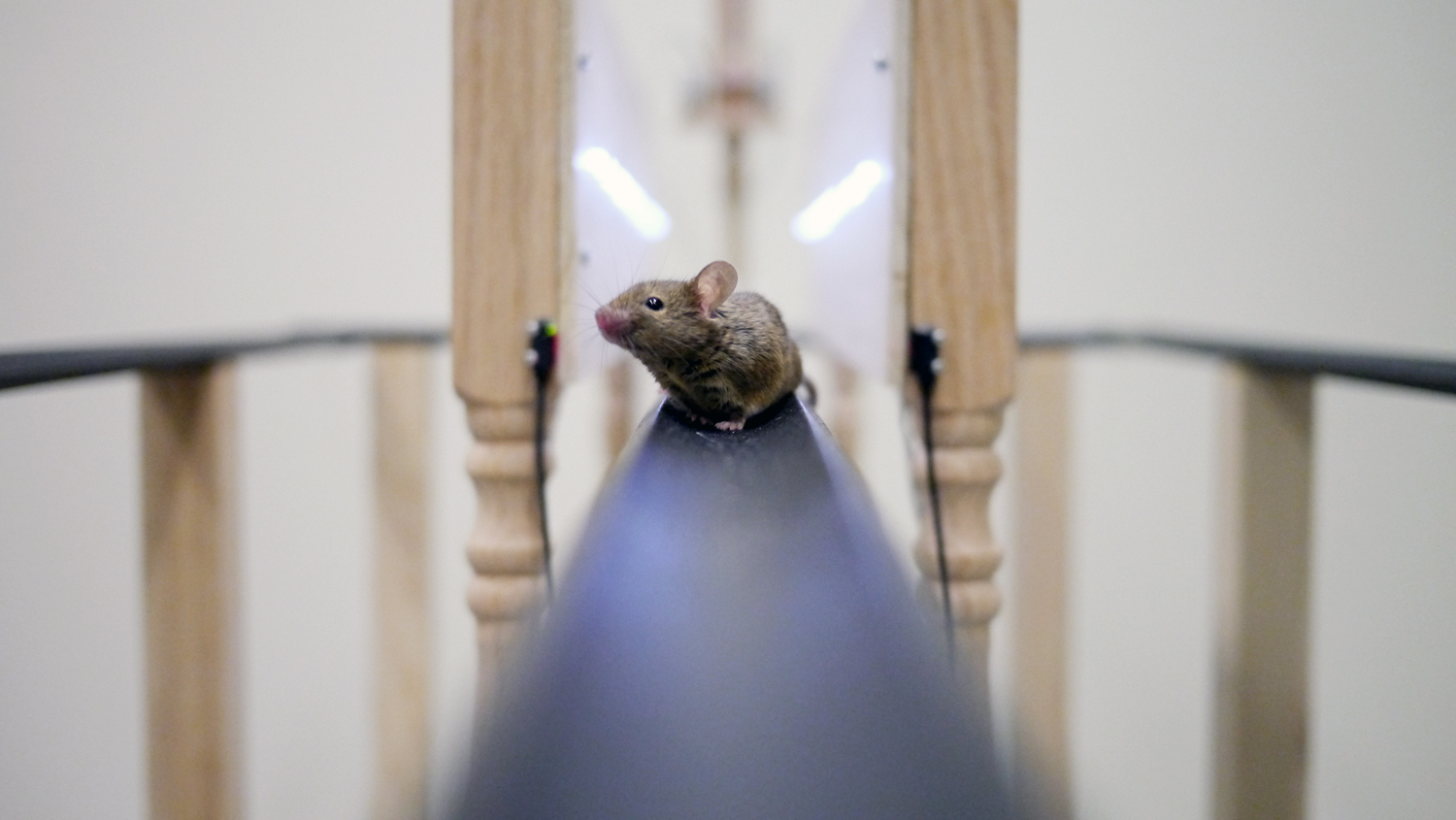
Currently, we are performing the following projects.
(1) Quantifying dynamics of monosynaptic-timescale connectivity in local neocortical networks during behavior
Can we quantify synaptic interactions between neurons in a local network in vivo? Are such synaptic interactions consistent during behavior, or do they change dynamically in a task-dependent manner? We are tackling these questions by using a high-density recording technique (64-channel silicon probe) in local circuits of the prefrontal cortex (PFC) in rats performing a working memory task.
In order to quantify fast neuronal interactions in the PFC local network, we developed a novel statistical tool to identify monosynaptic-timescale (~2ms) connectivity of neuronal pairs. We applied this method to the large-scale datasets, which enabled us to reconstruct the map of ‘monosynaptic’ interactions. Furthermore, we also quantified the dynamics of these monosynaptic-timescale interactions as a function of 'task phase', and we found the evidences that the strengths of the synaptic interactions changed dynamically in a task-dependent manner.
Taking advantage of these methods, we plan to reveal how the dynamics of functional synaptic connectivity relates to the implementation of the cognitive behaviors and learning processes.
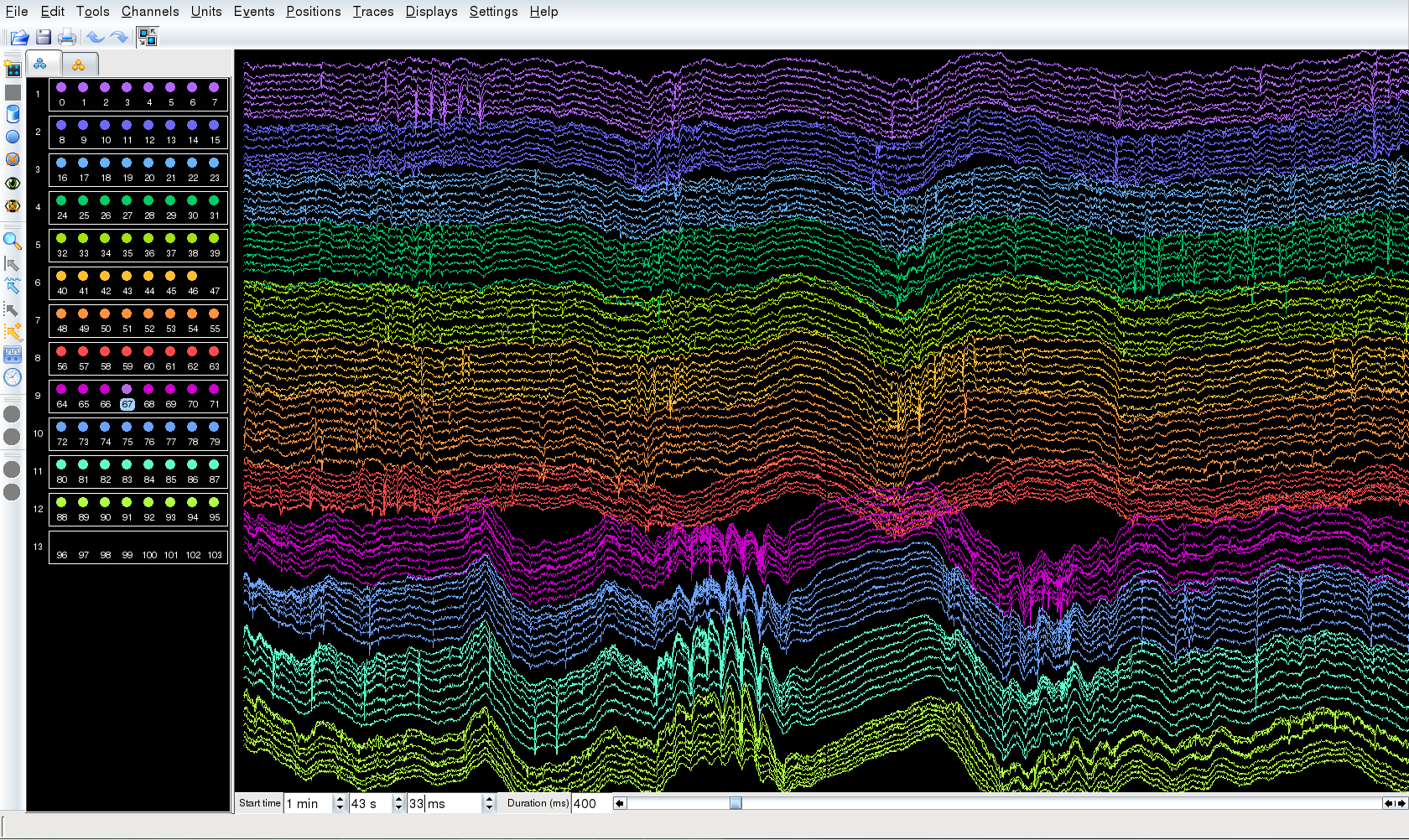
(2) A 4-Hz oscillation which synchronizes prefrontal cortex, midbrain VTA and hippocampus
Though dopaminergic inputs from the midbrain ventral tegmental area (VTA) are thought to have a critical role in the cognitive functions attributed to the prefrontal cortex (PFC), such as working memory, it is largely unknown how VTA dopaminergic neurons and PFC neurons might work synergistically on behavioral timescales.
Recently, we found a novel ‘4-Hz’ oscillation (2-5Hz) within the PFC and VTA, in rats performing an odor-based match-to-sample working memory task. Interestingly, this PFC 4-Hz and hippocampal theta oscillations (7-11 Hz) were phase-locked at their troughs. Neuronal activities and gamma oscillations in the PFC and VTA were strongly phase-locked to the 4-Hz oscillation. In particular, the goal-predicting PFC pyramidal neurons were more strongly phase-locked to both PFC 4-Hz and hippocampal theta oscillations than non-predicting cells.
These findings demonstrated that a 4-Hz oscillation is the dominant pattern in PFC-basal ganglia circuits during working-memory load, effectively modulating both local gamma oscillations and neuronal firing. Through phase-coupling, the 4-Hz and theta oscillations jointly coordinate neuronal assembly patterns in a task-relevant manner. This phase coupling between the 4-Hz and theta oscillators may be a mechanism for linking the entorhinal-hippocampal spatial-contextual system with the mesolimbic dopaminergic reward system.
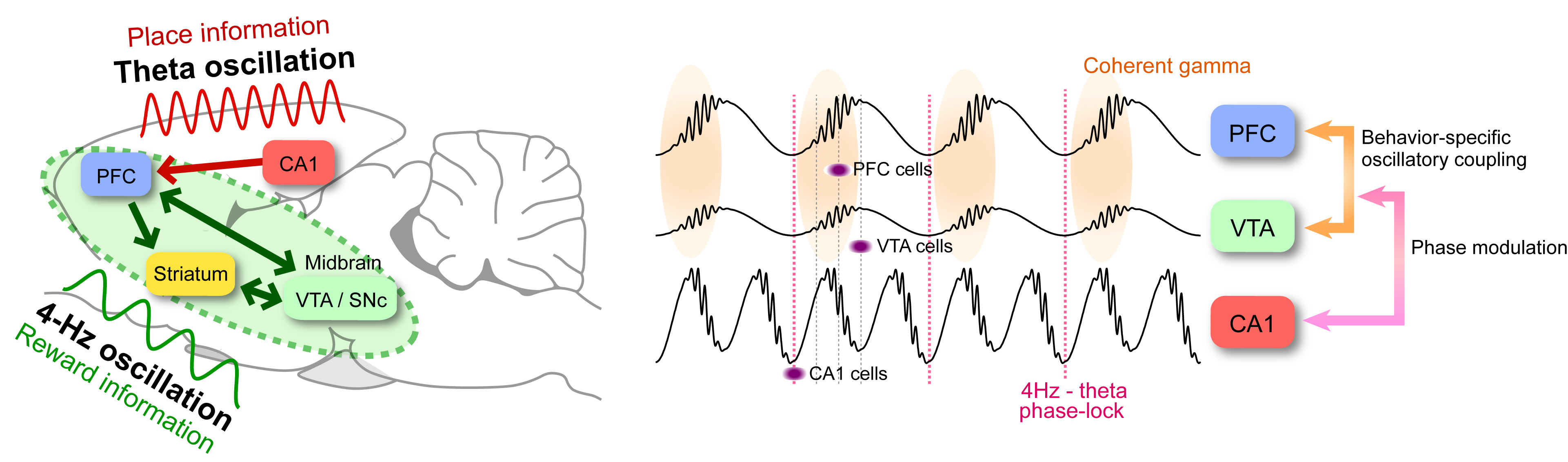
(3) Combining the techniques of large-scale extracellular recording and optogenetics
Recent advances in molecular optogenetical stimulation provide further tools for dissecting individual contributions of neuronal types and subpopulations to the dynamics and function of these circuits. We are currently developing combined techniques of large-scale extracellular recordings and optogenetics, to understand the functional dynamics of neuronal microcircuits during reward-related cognitions.
For example, a novel "optoprobe" with an array of laser-diodes has been developed recently, for the purpose of precise spatiotemporal optical control of multiple neurons in freely-moving animals. This is a 4~8 shank silicon probe with an optic fiber ~100 µm above the recording channels on each shank. Each optic fiber is connected with a laser-diode which can be controlled separately, allowing for local and precise spatiotemporal light stimulation of neuronal activators and silencers and the concurrent recording of the stimulated neurons. The system can be used to generate unique neural activity patterns not possible with other electrophysiological or optogenetic techniques.
By using we are building a close-loop for simultaneous recording and stimulation system, which can monitor multiple single neuronal activities and animals behaviors online, and enables real-time optical stimulations based on those physiological and behavioral parameters.
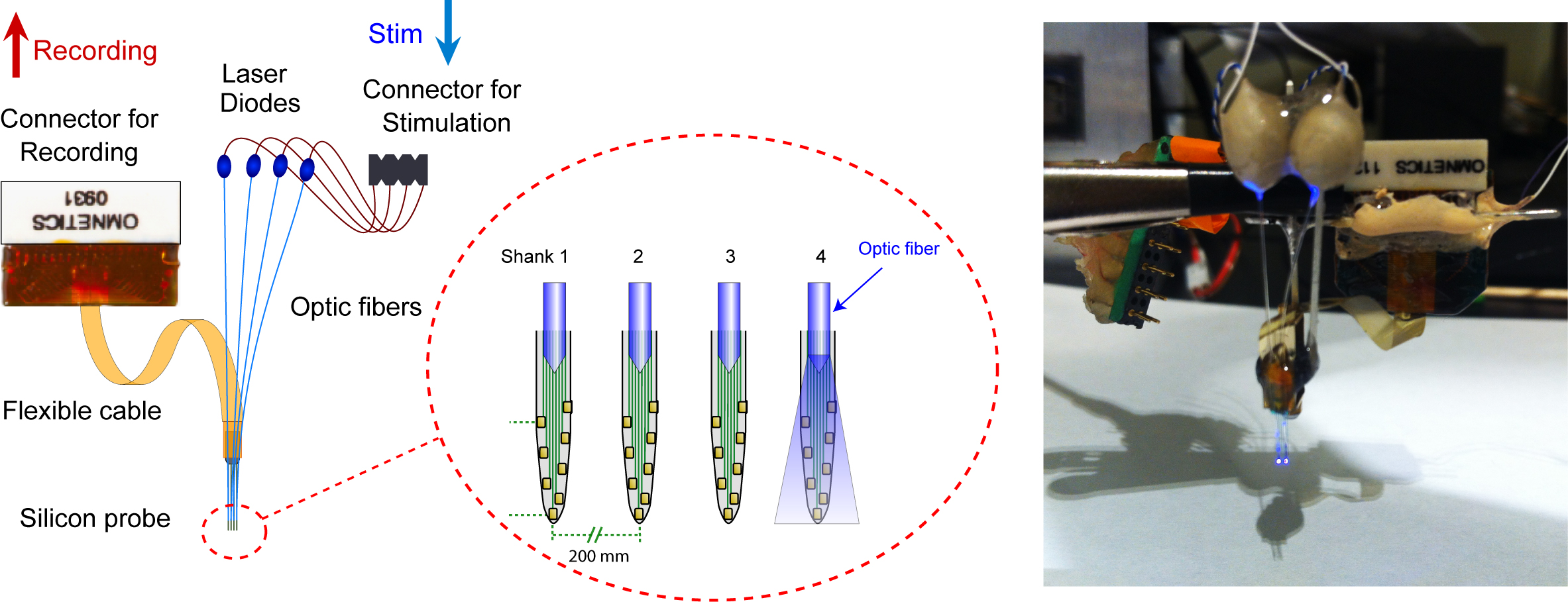